Take you away from the veil of strong interaction
Author:Institute of Physics of the Ch Time:2022.09.26
| Author: Dong Xiangkun 1,2, † Zou Bingsong 1,2,3, ††
(1 Institute of theoretical Physics of the Chinese Academy of Sciences)
(2 School of Physics Science, University of Chinese Academy of Sciences)
(3 Institute of Modern Physics, Chinese Academy of Sciences)
This article is selected from "Physics" No. 9, 2022
As one of the four basic interactions, the study of strong interaction can be traced back to the natural radioactive discovery of elements at the end of the 19th century. For nearly a century, after the unremitting efforts of many scientists, the veil of strong interaction was gradually revealed. The article will briefly introduce the Nobel Prize related to interaction with strong interaction, and use this as a carrier to sort out our cognitive process of interaction in strong interaction in the process of exploring the world.
Keywords strong interaction, sub -atom structure, strong sub -structure, Nobel Prize
01
Material interaction and its cognitive age
The current physics believes that there are 4 basic interactions in our world, namely gravity interaction, electromagnetic interaction, strong interaction, and weak interaction. Due to the different scale of interaction, these four basic interactions are discovered differently, as shown in Table 1. In 1687, after a long study and drawing on the experience and achievements of his predecessors, Newton proposed the law of gravity in his book "Mathematics Principles of Natural Philosophy", successfully explaining the sports trajectory of the planet. In 1915, Einstein proposed a broad sense of relativity, giving the essence of gravitational geometry. In 1785, Kulun gave Curon's law that describes the interaction between the two points of charge in the "Law of Electricity". Curon's law is the first quantitative formula in the electromagnetic phenomenon. After subsequent experiments and theoretical development, Maxwell summarized the Maxwell equations in 1865 and completed a unified description of electrical and magnetic. Almost all the phenomena we encounter in our daily life can be described in these two interactions, but by the 20th century, we gradually encountered the phenomenon that these two theories could not be explained -atomic nuclear structure and neutron decay. The study of these two issues enables us to recognize the existence of strong interaction and weak interaction. In 1934, Tangchuan Hideki and Fermi proposed the theory of media exchange theory and the interaction theory of four -expenditure. After subsequent development and improvement of these two theories, they eventually developed into the theory of quantum color dynamics (QCD) and power weakness in the 1960s and 1970s, forming the cornerstone of standard models.
Table 1 The first four basic interactions and the age of its discovery
In the process of strong interaction development, a large number of scientists have done many milestones and won the highest honor in the scientific community -the Nobel Prize. In Section 2 and 3, we will introduce the Nobel Prize related to interaction with strong interaction, understand the basic content and significance of these tasks, and clarify the history of we explore the micro -structure of the Asian atom. Section 4 We will briefly introduce the research on the frontiers of strong interaction.
02
Asian atomic structure
2.1 Atomic nucleus
Ernest Rutherford (1871-1937) won the 1908 Nobel Prize in Chemistry for the study of element decay and radiation chemistry. Lutherford is known as the father of atomic nuclear physics, radiochemistry, and atomic physics. Ludaseford was born in a farmer family in New Zealand. After obtaining a bachelor's degree in literature, a master's degree in literature and a bachelor's degree in New Zealand, he entered the Cavendish Laboratory of Caven University in 1895 and became a student of Joseph John Thomson. Tom Sun won the 1906 Nobel Prize in Physics for Electronics. In 1898, under the recommendation of Tom Sun, Lutherford went to McGill University in Canada to teach. In 1907, Lutherford returned to Britain, taught at the University of Manchester and returned to Cambridge in 1919. He took over as Tom Sun and became the fourth leader of the Cavendish laboratory. Lutherford is an outstanding experimental physicist. He treats each discovery with a rigorous attitude and makes the discovery including α, β -rays, the decline of elements, the launch of α particles, and the nuclear model of the atom. A series of significant results such as proton discovery and other significant results can be referenced [1]. In addition to his extraordinary scientific achievements, Lutherford is also a great scientist. Among his students and assistants, 10 have won the Nobel Prize. This is a achievement that is forever and cannot be achieved.
2.1.1 α, β -ray discovery
In 1895, German scientist Lunqin unexpectedly discovered a new ray -X -ray when he studied cathode rays, and X represents unknown. This discovery, especially the photos of Mrs. Lunqin's hand bone, caused a great sensation once it was announced -X -rays were like a pierced magic. Ludaseford conducted a study of X -ray electric ionization at the suggestion of Tom Sun, which was a major popularity of physical research at that time. In 1896, French scientist Berchler discovered the natural radioactivity of uranium, and Luuseford turned to uranium radiation research after learning about it. He found that in the radiation of uranium, there are two different components of penetration. In 1899, he was called α rays that were easily absorbed by uranium radiation, and another ray with stronger penetration was called β -ray [2]. Although early experiments found that these rays were not deflected by the electromagnetic field, that is, electrical neutrality, the subsequent experiments of Lutherford showed that the α -ray band positive electricity and the quality of the hydrogen atomic at a magnitude. Lutherford believes that α -rays are two positive charged ions, but it was not until 1908 that he provided conclusive evidence -the spectrum of α -rays and the spectrum seen when he found in the sun in 1895 The same [3]. In addition, he also called the third radiation of uranium discovered by French scientist Villa in 1900 as γ -rays. These names are still standard. 2.1.2 Radioactive transformation
After coming to Canada, Lutherford continued to conduct radioactive research. In 1900, when Lutherford studied the ionization properties of the 钍 (atomic order Z = 90) radiation, he found that the gas around the 钍 was also radioactive, and Lutherford was called a pupa. He was very confused about the chemical nature of this radioactive gase. This year, a young British chemist Sudy entered Lausford's laboratory to identify the essence of these radiation gases. In the end, they confirmed that the change of 过 after experiment was
They sorted out these experiments results and got a seemingly incredible conclusion at that time. -Elements will become other elements after release of rays. For a long time, everyone thinks that atoms, as the basic elements that constitute the material world, are stable. Ruudford's experimental results challenged this concept and won the 1908 Nobel Prize in Chemistry.
2.1.3 The nuclear model of the atomic
After discovering the electrons, Tom Sun proposed the atomic raisins -pudding model -the positive charge was evenly distributed in the entire atom and the electrons were inlaid like raisins in pudding. Ruudford originally agreed with this model. "Moving electrons, that is, components that constitute atoms, need to be tied up together." After Ludaseford studied α particles, he decided to use it with The α particles bombard the gold foil to see its scattering distribution, and then verify this atomic model -either all α particles can easily pass through the gold foil, and there is almost no bias. (A) Show.
Figure 1 Tom Sun Model (A) and Lumosford Model (B) α -particle schematic diagram (picture is taken from the Wikipedia entry "Rutherford")
In 1908, Ge Ge got preliminary results under the guidance of Lutherford. They found that, as expected, all α particles passed through gold foil, and they were concentrated in small scattered corners. In the second year, Masden, another student of Ge Ge and Lutherford, continued to study α -scattered experiments. Lutherford asked Muson to see if he would detect α particles at a large angle. The result of Masden -about one thousandth of α particles will have a large angle scattering -shocked Lutherford, "This is the most incredible thing I encountered in my life. It's as if you use one with a one The 15 -inch cannon bombard a piece of paper and you have been hit by the shell that rebounded back. [5]. "In order to explain this phenomenon, Lutherford proposed the nuclear model of the atom -atom with a positive electrical nuclear carried atoms with an atom with the electrical electrical nucleus carried atoms with an atom with the electrical electricity. Most of the quality only occupy a very small area in the center, and most of the peripherals are empty, as shown in Figure 1 (b). Lutherford derives the scattering section of this atomic model, and the Lurodford formula obtained can well explain the angle distribution of the α particles in the experiment [6].
The nuclear model of the atom is essential for subsequent understanding of the atomic structure. Based on this basis, Ruudford's student Bohr successfully explained the atomic spectrum proposed by the quantization conditions and promoted the establishment of quantum mechanics.
2.1.4 Find of protons
In 1919, when Lurodford bombarded nitrogen with alpha particles, he found a signal like hydrogen atom on the receiving screen. Lutherford speculates that the α particles have made the nitrogen nucleus into a carbon atomic and hydrogen atomic nucleus.
Lutherford determined that this process was the first artificial element conversion process -high -energy alpha particles could make a nucleus with a smaller quality atomic nucleus [7,8]. This end -of -the -tape charged particles similar to hydrogen atoms are called proton 1 by Lutherford).
After discovering the proton, Lutherford proposed the concept of neutron. At that time, people thought that the various atomic nucleus seen in the experiment were composed of electricity with a charged hydrogen atomic nucleus, such as N-14 consisting of 14 hydrogen nucleus and 7 electronics. Lutherford believes that protons and electrons in the atomic nucleus may form a neutral deep restraint, namely neutron. In 1932, the atomic nucleus neutralized particles predicted by Lutherford were discovered by his student Chadwick.
2.1.5 Great Academic Instructor
Frederick Soddy (1877-1956) won the 1921 Nobel Prize in chemistry for radio chemistry and research on the essence of isotropic. Sudy followed Lumosford's radioactive research from 1900 to 1907, and found that radioactive substances can be turned into other elements by release radiation lines (1). In 1913, he found a radioactive substance, even if he had exactly the same chemical properties, he could have different quality numbers. Sudy called them homogeneous [9].
Francis William Aston (1877-1945) won the 1922 Nobel Prize in Chemistry for using a large number of non -radioactive elements and the proposed "WHOLENUMBER RULE". Aston and Lutherford were both students of Tom Sun. The two spent a lot of time in the Cavendish laboratory and became very close colleagues and friends. After the First World War, Aston returned to the Cavendish laboratory to improve the instrument that identified particles at the time and improved the resolution of quality [10]. Aston has greatly expanded the research of lubble and Sodilicon's development of homogenous research with his own mass spectrometer, studied about 30 kinds of non -radioactive elements, and accurately measured their quality. Hydrogen atomic quality. These discoveries have promoted people's understanding of the nucleus structure.
Niels Bohr (1885-1962) won the 1922 Nobel Prize in Physics for the study of atomic structure and radiation. In 1912, 26 -year -old Danish physicist Bohr was invited to come to the University of Manchester to follow Lumosford for a postdoctoral research. Based on the nuclear model of Lutherford's atomic nuclear model, Bohr proposed the planetary orbital model [11], that is, peripheral electrons can only turn around the atom nuclear rotation around the atomic nuclear rotation at a specific frequency. Electronics can jump between different tracks, and then absorb or release electromagnetic waves with corresponding energy. The reason why Bohr put forward this intuitive difficulty to understand is to solve the fatal lack of fatal in the Ruuseford model -the charged particles are used as a circular exercise to release the energy of electromagnetic wave loss, which means that all atoms are unstable. It is seriously inconsistent with reality. At the same time, this semi -classic rail model can also explain the discrete spectrum of atoms and promote the establishment of subsequent quantum mechanics.
Charles Wilson (1869-1959) won the 1927 Nobel Prize in Physics because of the invention that can show the cloud room with a diameter of charged particles. In 1896, Wilson, who worked in the Cavendish laboratory with Lutherford, invented the clouds and fog. In a closed container, enter pure ethanol or methanol steam to reduce the steam by reducing the temperature. If there is a charged particle to enter, an ion will be generated on the path. The excessive saturated vapor will be condensed into a small drops with ions as the core, which shows the diameter of the particles. The study of X -ray electric ionization under Tom Sun's suggestion, which played an important role in the mechanism of understanding the cloud room 2).). After Lutherford discovered α and β -rays, Wilson first observed and photographed the trails of α and β particles with a cloud room.
James Chadwick (1891-1974) won the 1935 Nobel Prize in Physics. Chadwick has studied work with Ruuseford since undergraduate and obtained a master's degree in 1913. After the war, he studied his doctorate under the guidance of Ruusefufu and worked as a Ludaseford's assistant at the Kavadi laboratory. After more than ten years. In 1930, German physicist Walther Bothe and the student Baker bombarded the α particles radiated by 钚, and found an unknown ray. In 1931, the French Settle and Irène Joliot-Curie also observed this neutral ray and they mistakenly believe that it is high-energy photon. Ruudford and Chadwick did not agree with this view, and they thought it might be a neutron predicted by Lurodford before. Chadwick launched a neutron detection and verification experiment in overtime. Just two weeks later, Chadwick sent his papers "Perhaps the Middle School" [12] to Nature magazine.
Oto Hayn (1879-1968) won the 1944 Nobel Prize in Chemistry for his discovery of nuclear fission. From 1905 to 1906, Harne worked as a assistant at McGill University as a assistant in Lutherford. During this period, he discovered that several radioactive elements were homogeneous. In 1939, Harne and his student Fritz Strassman used neutron to stimulate the uranium nucleus to detect 钡 [13] at the end of the state. This is completely different from the α, β, γ decay observed before. Later, the theoretical studies of Lise Meitner and OTTO Frisch found that the uranium nucleus had split into a lighter nuclear [14, 15]. This fission phenomenon was critical to the use of subsequent atoms.
Patrick Blackett (1897-1974) won the 1948 Nobel Prize in Physics because of the improvement of Wilson's cloud room and studying a large number of particles in cosmic rays and nuclear reactions. Blacht has worked in Lubay's laboratory for ten years since 1921. In 1925, he used the cloud room to prove that the real process in Luuseford's discovery of proton experiments was (3) instead of (2) [16]. In 1932, Blacht connected Wilson's cloud room with the Ge Ge counter, and used this improved detector to discover a large number of particles in cosmic rays, including verifying the existence of positive electrons, observed the generation of positive and negative electrons, Wait a series of important results. John Crovt and Onest Walton (John Cockcroft (1897-1967 ERNEST WALton, 1903-1995) obtained a pioneering work of the nucleus of artificial accelerated particles to the atomic nucleus. Nobel Prize in Physics in the Year. Kokrovt and Walton are students from Lutherford's laboratory in Cavendish. After Lutherford's laser radiation α particles bombarded nitrogen nucleus to get the proton, he hoped to get higher energy particle beam bombardment of the atomic nucleus to get more reaction processes to explore the nucleus structure in detail. Lurodford arranged this task to Kokrovt and Walton and others. In 1932, Kokrovt and Walton successfully built a DC high -voltage accelerator to replace the natural radiation alpha particles beam flow. For the first time, the nuclear reaction generated by artificial acceleration particles was achieved, and the new world of particle physical experiments opened up.
Pyotr Kapitsa (1894-1984) won the 1978 Nobel Prize in Physics in the field of low temperature physics. After World War I, Carpicha came to the UK and worked under Ludaseford for 14 years and became the newly established Monde Laboratory director in 1930 to study the magnetic field. He returned to the Soviet Union to visit his relatives in 1934, but the Soviet government did not allow him to return to Britain again. Lutherford wrote to the Soviet government to negotiate without results. Carpizha decided to change the direction and conduct low -temperature physics research. With the help of Lutherford, he purchased the experimental instruments of Monde's laboratory and established the Institute of Physics.
2.2 neutron physics
Enrico Fermi (1901-1954) was won by the 1938 Nobel Prize in Physics for using neutron radiation to discover new radioactive elements and discovering slower neutron reactions. Fermi is a rare physicist who has made outstanding contributions in both theoretical and experiments. In terms of theory, his contribution is concentrated in the fields of statistics, quantum physics, nuclear physics and particle physics. In terms of experiments, he used neutron radiation to induce nuclear reactions, lead the "Manhattan Plan" of the world's first artificial nuclear reactor, and participate in the American construction of the atomic bullets in the United States.
Since Nako was discovered by Chaderk in 1932, it has become a new tool for studying nuclear structures. In 1934, people found that some atomic nuclear absorption of α particles would be radioactive. Fermi decided to replace the α particles for neutron. The neutron does not charge, and it can be captured by the atom without having to overcome the potential energy of Kulun, which in turn induces nuclear reactions. After a series of neutron -induced verification experiments, the Fermi Experimental Group found that the energy brought by the light atomic nucleus will be taken away by the linked protons or α particles released. Essence According to the theory at the time, after the nuclear capture of the neutrons, the β decay occurred, Z will increase by 1. When they bombarded the uranium nucleus, they did observe this phenomenon -the half -life of many particles at the end of the state was different from the original nucleus known at the time. So they claimed to discover elements No. 93, 94. Because they failed to separate these new elements, their discovery was questioned by some scientists. Nevertheless, the Nobel Prize Committee awarded the 1938 Nobel Prize to him because of Fermi discovered two new elements. One month after the Nobel Prize was promulgated, Hayne and others discovered the phenomenon of fission of the nucleus [13]. The two "new elements" claimed by Femomey are proved to be the product of nuclear fission, both of which are homogenin of known elements.
Afterwards, although the Nobel Prize Committee's reasons for the award for Fermi were wrong, no one would question the rationality of Fermi to win the Nobel Prize -he did Nobel Prize -level work in many aspects. In the 1920s, the energy of the end -of -end electrons during the decay of β was undoubted. To understand this matter, Paoli assumes that in addition to protons and electronics, there is also a very small particle of electrical neutrality in addition to protons and electronics, and it is called "neutron". Fermi accepted this view and changed its name to "neutron". Fermi also constructed four -expense Miko interaction to describe β decay. The theory has gradually evolved into the theory of weak interaction in standard models after subsequent development of scientists including Li Zhengdao and Yang Zhenning. In the field of statistical physics, the particles of spinning into a half -integer are named after his name, that is, Fermiko. He analyzed the ideal gas system (that is, Fermi Gas) composed of Fermiko, and used the statistics of Fermi -Dirac to describe their status distribution. In the field of quantum physics, Fermi's gold design describes the transition rate between different energy signs. For these kinds, Fermi's development of modern physics is important. 2.3 Nuclear Power and atomic nuclear structure
Hideki Yukawa (1907-1981) was won by the 1949 Nobel Prize in Physics for the existence of π meson because of theoretical analysis of nuclear force. After the neutron was discovered in 1932, people gradually realized that the atomic nucleus was composed of proton and neutron (collectively referred to as nuclear). However, the proton belt is positive, and the neutron is not charged. They cannot form an atomic nucleus together through electromagnetic interaction. Fermi is very weak in describing β decay, which is not enough to restrain the nuclear at all. [17] In 1934, Tangchuan Xiushu introduced a new interaction to explain the attractiveness between nuclear [18]. Similar to the electromagnetic interaction through the exchanging photon, the nuclear interaction is achieved by switching the meson, and the restraint is generated. According to the size of the nucleus, the Tangkawa Hideshu, R ~ 1 FM (~ 10-15M), is estimated that the quality of Jieon is about M∼1/R∼100 MEV.
Cecil Powell (1903-1969) won the 1950 Nobel Prize in Physics for the development of latex photography methods for studying nuclear reactions and using this method. After Tangchuan Hideki predicts the π meson that transmits nuclear power, physicists began to look for it. In 1936, Miao Zi (发现) was discovered that its quality can reach 150 MEV, which is very close to the predictions of Tangchuan Xiushu, so at the beginning, it was thought that this was π meson. But subsequent experiments found that Miao Zi did not participate in nuclear reactions. From 1939 to 1942, two Indian scientists Debndra Mohan Bose and Bibha Chowdhuri studied cosmic rays in the high altitude area of India with photography. [19,20]. They observed particles with a quality of about 200 times electronic quality in cosmic rays. In 1947, Powell and others improved this method and observed this meson in cosmic rays [21]. They also found that this meson participated in the interaction of nuclear, and further support it as the π melonon of Tangchuan Xiushu.
Eugene P. Wigner (1902-1995) won the 1963 Nobel Prize in Physics for the discovery and application of the principle of basic symmetry, especially the principle of basic symmetry. Vogner has made important contributions in the field of theoretical physics and mathematical physics. He applies symmetrically in physics research, and many theorems and concepts are named after his name, including Wigner -Eckart theorem, Vogner theorem, Vogner classification, Vogh Na D-function, Vigner 6-J, 9-J coefficient and so on. In the 1920s, Vigner introduced symmetry into the newly established quantum mechanics, which obtained a series of important results. In the 1930s, Vigner discovered that the interaction between nuclear was very weak when it was far away, and it would become stronger when approaching. He also noticed that the symmetry of nuclear satisfaction did not distinguish the molecular and neutrons, and then the strong interaction that restrained the nuclear together was the same to the proton and neutron.
Maria Goeppert Mayer (1906-1972 Hans Jensen, 1907-1973) won the 1963 Nobel Prize in Physics. After people recognize that the nucleus is composed of protons and neutrons, an important problem is the relationship between the various properties of the nucleus and the number of neutrons and the number of neutrons. Due to the lack of precise theory of describing nuclear force, people proposed the phenomenon model to describe the nature of the atomic nucleus based on the experimental results. The initial proposed liquid droplet model assumes that the nucleus is formed by the propyl of the proton and the neutrons under the common action of electromagnetic and nuclear force, and the energy of the entire droplet is on the surface tension, volume, Kulun force, asymmetric, and nuclear pairing. Wait for 5 aspects. As a result, Bethe -Weizsäcker Mass Formula can describe the nature of the atomic nucleus well, but it cannot explain the existence of "Magic Number". The experiment found that the atomic nucleus with certain proton or neutron numbers was relatively stable. Vigner called these numbers as "fantasy", including 2, 8, 20, 28, 50, and so on. To explain this phenomenon, in 1949, Meyer and Yansen independently proposed the shell model of the atomic nucleus [22-24]. Similar to the arrangement of electrons in the atom, the proton and neutrons in the nucleus also have different energy levels. Whenever a energy level is filled, the atomic nucleus is relatively stable. Aage Bohr, 1922-2009, Ben Mottelson, 1926-2022 Leo Rainwater, 1917-1986) The connection between the relationship and the development of the atomic nuclear structure based on this connection won the 1975 Nobel Prize in Physics. The shell model proposed in 1949 explains the phenomenon of the "magic number" of the nucleus, but the electrode teta of the nucleus obtained does not match the results of the experiment. In 1950, the Renwater of Columbia University speculated that the nucleus was a whole, and its shape would be influenced by each internal nuclear movement without the symmetry of the ball [25]. A. Bohr visited the University of Columbia at the time. After communicating with Rynewater, he more generally studied this issue and discussed the impact of the movement of a single nuclear on the overall movement of the atomic nuclear. A. After returning to Copenhagen, Bohr compared the theoretical and experimental results with Mot Song, and found that their models were consistent with the experimental results, and the shell model was linked to the concept of Renwart's atomic nuclear change [26— 28].
2.4 Georitic nuclear physics
Hans Albrecht Bethe (1906-2005) won the 1967 Nobel Prize in Physics due to the development of nuclear reaction theory, especially with nuclear fusion reactions. Bate is a well -known German American nuclear physicist. After the Nazi in power in 1933, Bett was fired. He immigrated to the United States in 1935 and became a professor at Cornell University. In 1938, Bett was invited to attend a seminar on "how to generate star energy". He had no intention of attending this meeting that he was not interested in, but Edward Teller persuaded him. At the meeting, Bate learned about the temperature, density, elemental composition of the sun. Then Bett proposed two nuclear reaction processes to explain the source of the sun [29]: One is that the two hydrogen nucleus becomes a nucleus, and the nucleus further captures the proton into 4HE to release the energy; For the catalytic of nitrogen nuclei, the four hydrogen nuclei became 4HE and released energy. They are called hydrogen -P -P -P -P -PHAIN and carbon -nitrogen -oxygen cycles (CNO Cycle), as shown in Figure 2 (a), (b). After calculating Bette, the proportion of these two processes is roughly the same near the temperature of the sun. The above nuclear reaction process proposed by Bett successfully explained the source of the stars' energy.
Figure 2 The hydrogen-hydrogen chain (a) and carbon-nitrogen-oxygen cycle (b) process in the star (the picture is taken from the Wikipedia entry "Proton-ProTon Chain" and "CNO Cycle")
As a well -known nuclear physicist, Bate has also done many other important tasks, including Bethe Ansatz, which is parameterized by the quantum multi -system system. The explanation of Bethe Bible, Lamb Shift, the relativity equation of the two bodies -Salpeter Equation, which is a relatively contented equation -Salpeter equation. Bett published his first academic paper from the age of 18, until he was still in his 90s, and even a cooperative article was published after his death. His former post -doctoral Freeman Dyson called him "an important problem solvers in the twentieth century."
Figure 3 Cosmic Element Enlightenment Curve [30]
William Alfred Fowler (1911-1995) won the 1983 Nobel Prize in Physics for the theory and experimental study of the nuclear reaction of chemical elements in the universe. The stars in the universe are formed by gas and dust clouds. When they are pulled together by gravity, gravity potential can be released in the form of heat. When a high temperature is reached, reactions start between the atomic nucleus inside the star. These reactions are the reason for the glowing of the star. On the other hand, in the 1950s, people had measured the distribution of cosmic elements (in fact, the abundance of elements in the sun), as shown in Figure 3. From the figure, we can see the following characteristics: the index of elemental abundance decreases with the increase of the quality of the atom; the slope is reduced rapidly after the quality of the atoms is greater than 100; The significant peaks near 56FE; some twin peak structures and so on. How is these elements formed by the initial hydrogen? Why do these trends and characteristics shown in the figure appear? In the famous B2FH articles [30], Fortevi and others have proved that the nuclear reactions in the stars can explain these characteristics of the element abundance curve, which greatly promotes our understanding of the formation of elements in the universe. 2.5 Nuclear Technology Application
Felix Block (Felix Block, 1905-1983 Edward Mills Purcell, 1912-1997) received a new method for developing MRI's measurement, effectively studying the composition of various materials and obtained 1952 Nobel Prize in Physics in the Year. The proton and neutrons in the nucleus are like small, rotating magnets, so the atoms and molecules are arranged in the magnetic field. Electromagnetic fields can disturb their direction, but according to the principle of quantum mechanics, they can only follow a specific direction. When the nucleus returns to the original position, they will emit electromagnetic waves of a specific frequency, which depends on the type of element. In 1946, Periser and Bloch developed accurate measurement methods to study the ingredients of materials. This method has now developed into a very mature technology and has been widely used in all aspects of production and life.
Bertram N. Brockhouse (1918-2003) (1918-2001) Learn award. In the 1940s and 1950s, with the completion of many nuclear reactors, the radiated neutron provided people with a new probe for researching the material structure. Different from X -rays, non -electricity neutrons do not be affected by electrons in the material and can reach the atomic nucleus directly. On the one hand, when the neutron and the material collide with the atomic nucleus, the energy of the neutron is converted into the vibration of the lattice. These vibrations called Shengzi have a fixed energy level, forming a series of energy spectrum. In the 1950s, Brockhaus developed technologies that analyzed different molecules and material attributes using these spectra. On the other hand, according to the principles of quantum mechanics, neutron and other particles can be described as a wave of movement, and neutron radiation can generate specific diffraction patterns through regular atomic structures. In 1946, Schale developed a new method to use this to determine the structure of different molecules and materials. Neutral spectrum and neutron diffraction technology have played a great role in subsequent research on condensation physics and material science.
03
Quark and quantum color dynamics
3.1 proton structure
Orto Stern (1888-1969) won the 1943 Nobel Prize in Physics for the contribution of the molecular ray method and the discovery of the proton magnet. As an experimental physicist, Stern has made many famous experiments, including one of the most famous Schuton -Grah experiments -one of the foundation of quantum mechanics. Stein -Grah experiment confirmed the quantization of atomic angle momentum, but its experimental phenomenon did not be fully understood until the concept of electronic spinning of electronic spin until G. Uhlenbeck and S. Goodsmit proposed the concept of electronic spin. Essence The spin of the electron is 1/2, and its magnetic torque is
G is Lange G factor. Electronics is the basic particles, and the high -level correction of electromagnetic interaction is not considered, g = 2. If the proton is also a basic particle and has no internal structure, the theory predicts that its magnetic torque should be μP≈ 2.5 μN. This means that the proton has an internal structure and cannot be basic particles.
Robert Hofstadter (1915-1990) was founded in a pioneering study of electron -1990, and found the nuclear structure in 1961. Since being discovered, whether the nuclear has been a basic particle has always been a problem that everyone cares about. The proton magnets obtained by Stern were severely deviated from the dot particles, indicating that the proton has an internal structure. Hofstatt used the electronic -proton scattering experiment to measure the size of the proton about 0.7-0.8 FM, which further confirmed that the proton was not a dot particle (Figure 4) [31].
Figure 4 Electronic energy is 188 MEV. The electron -proton micro -scattered scattering section changes with the scattering angle [31]. Assuming proton is a dot particle, the theoretical results are shown in the curve C, and there is a deviation from the experimental data 3.2 Quark model and quantum color dynamics
Donald Arthur Glaser (1926-2013) won the 1960 Nobel Prize in Physics for the invention of the bubble room. The clouds invented by Wilson can let us observe the trace of charged particles. The bubble room invented by Graze in 1952 allows us to study more energy particles. When the charged particles pass forward through the liquid room full of near the boiling point, the atoms they pass will be separated. When the indoor pressure is reduced, air bubbles will appear around these ionizing atoms, and then we can take pictures and analyzes the trajectory of the particles. The invention of the bubble room has made people detect more particles and has made important contributions to the research of strong sublates.
LUIS Walter Alvarez (1911-1988) (1911-1988), especially for the decisive contribution of basic particle physics, especially developed the technical and data analysis of the use of hydrogen bubble rooms, found a large number of resonance states, and obtained the 1968 Nobo in 1968 Bell Physics Award. Following the bubble room of Graze, in the second half of the 1950s, Alvarez further developed the bubble room by using liquid hydrogen. He also developed a new measurement system and computer -based method to analyze a large amount of data, which found a large number of unknown particles before.
Murray Gell-Mann (1929-2019) won the 1969 Nobel Prize in Physics for the classification (quark model) and its interaction. In the 1950s and 1960s, with the application of bubble rooms, hydrogen bubble rooms, and particle accelerators, people found a large number of "basic" particles. As of 1963, the particle data table [32] has included nearly a hundred "basic" particles, including many unstable resonance states. It is difficult for people to believe that all these particles are basic particles. Before Garman proposed the quark model, people tried to understand the internal structure of these "basic" particles and classified them, including the Sakata model in 1956 and the eight -tier proposed by Galman and Yuval Ne'eman in 1961 in 1961 Eightfold Way.
In 1964, Georgezweig, a predecessor's study, independently introduced Quark 3) such a more basic particle to describe the strong son found in the experiment. Quick carries the foundation of the SU (3) group (3), with three flavors in total, namely the upper (u), the lower (d), the strange (s) quark.
SU (3) The reputation of the group foundation
Table 2 quantitative quantum number, from top to bottom, is spin, charge, charge, equivalent, equivalent rotation, 3rd components, strange numbers and weights
Samuel Chao Chung Ting Ting (1936 -Burton Richter, 1931-2018) won the 1976 Nobel Prize in Physics. While the research and development of atomic nucleus and Qiangzi, people's understanding of weak interactions has gradually deepened. By the 1960s, the theory of Higgs mechanism and power weakness was established. In 1970, in order to explain the existence of a neutral flow that changed the serious taste of the experiment, Grace, Illiopolos and Sheldon Glashow, John Iliopoulos Luciano Maiani) proposed the GIM mechanism and introduced a new quark 4 4 ) - —— 粲 粲. In 1974, Ding Zhaozhong and Ricker found a very narrow resonance state in the proton -nuclear collision and positive electronic collision, respectively, with a mass of about 3.1 GEV. Ding Zhaozhong and Ricktn named it J particles and ψ5 respectively). Because its quality is far greater than the strong son of the previously discovered, the width is very small. It cannot contain only U, D, or S quark's strong son. Therefore Essence C Quark's discovery has extremely significant significance, known as the "November Revolution" -it tests the correctness of the theory describing weak interactions and strong interactions at the time. The other two flavors, the heavier Quick, the bottom quark (b) and the top quark (T) were also discovered in 1977 and 1995, respectively, and completed the puzzles of the quark part of the standard model.
Jerom Frendman, Henry Kentor and Richard Taylor (1930-, Henry Kendall, 1926-1999 and Richard Taylor, 1929-2018) The pioneering research of the deep non -elastic scattering of the child then confirmed the existence of Quark and won the 1990 Nobel Prize in Physics.
The quark model proposed by Garman and Zwog can classify the meson and heavy sub -discovered in the experiment, and put them in the multi -state of the SU (3) group. But soon people discovered new problems. On the one hand, is the quark of the basic component of the strong child? Is the real particle? Why didn't you see the free quark in the experiment? Or is Quark just as an auxiliary theoretical concept to facilitate understanding a large number of strong children found in the experiment? On the other hand, people found that in the Ten Chongzi, Quark seemed to violate the principle of compatibility of Paoli as Fermiko. For example, Δ ++, Δ-and ω- The quark group in the quark model is UUU, DDD and SSS, respectively. In the experiment, these particles were found as the base state. The total spin was 3/2 and the spatial wave function was both S waves. In this way, its overall wave function is exchanging all -symmetrical rather than Fermiko that needs to be satisfied. To solve the second problem, a degree of freedom called color (color) must be introduced. Each taste quark has three colors: red, green and blue (R, G, B), which carries the basis of another SU (3) symmetry. As long as the color wave function of the above three heavy sons is the single state of the color SU (3) group to meet the switching of the wave function. After the introduction of new color freedom, new problems followed. Why are the strong sons found in the experiment? To explain such a phenomenon, people have put forward the assumptions of color prohibition: only the single state of the color SU (3) group can exist freely. Because Quark is a triple state of color Su (3), we cannot detect free quarks in the experiment.
If you can't detect free quarks, do quarks really exist? The deep non -bullet scattering experiment in the 1960s gives a positive answer [35, 36]: There are point -shaped strip particles inside the nuclear. Electronic -proton deep non -bullet scattering has a high probability of large energy and large -volume transfer (Figure 5). This process of large energy and large volume transfer implies that there are scattering centers with domains inside the proton.
Figure 5 Electronics — Proton deep non -bullet scattering data [35]. The horizontal coordinates are the square of the metastasis, and the vertical coordinates are the ratio of the Kolun scattering section (Mot section) of the scattering section and the relativity particles. The experimental data shows that the cross -section of the large amount of motion transfer is not as fast as elastic scattering decreased quickly.
David Gross, David Polzee, and Frank Verchek (David J. Gross, 1941—, H. David Politzer, 1949 — and Frank Wilczek, 1951-) Won the 2004 Nobel Prize in Physics. Quantum color dynamics (QCD) is the basic theory of interaction in the standard model. It is a specified theory based on color SU (3) symmetrical. In 1973, Polyze, Gross and his student Verchek independently discovered the gradual freedom of the non -Abel norm field [37, 38], which shows the following forms.
Among them, αS represents the fine structure constant of strong interaction, that is, interactive intensity, B0 = 11-2nf/3, and m is any heavy positive energy point. When the number of quarks taste nf = 6, B0 & 0, at this time αS (Q) is zero as interaction can marked Q, which is the gradual freedom of QCD.
04
Strong Interaction Frontier Introduction
At present, the research on the research on strong interaction mainly includes three aspects: strong sub -structure, nuclear structure and high -temperature and high -secret nuclear substances under extreme conditions. These three fields account for about 30%of the relevant funds of the Ministry of Energy of the United States, and the remaining parts are allocated to related cross -disciplinary research, such as the inspection and nuclear technology application of nuclear body physics, standard models in nuclear physics, and the application of nuclear technology.
4.1 Structure
QCD is currently recognized as the basic theory of interaction that describes strong interaction. However, due to its low -energy non -micro -disturbance characteristics (the QCD coupling coefficient is no longer a small amount in the low energy zone, and it cannot be expanded by micro -disturbances according to its power), it is difficult for us to directly calculate from QCD strictly calculate the quarks. The spectrum of the child cannot accurately describe how the quark and the gluezi constitute a strong son. Compared with the atomic structure and the nuclear structure of the atomic, the biggest difficulty of studying the strong sub -structure is that the non -quenching effect of the component score is variable: the kinetic energy and potential energy in the strong child are greater than the quality of the positive and anti -Quick. Chengzheng Anti -quark
Figure 6 Traditional Qiangzi and Strange Strong Child State, QCD also allows more color single -state strong children of Quark components
At present, there are two basic ways to explore the experiments of the internal quark of Qiangzi -glue structure: one is to measure the quark -gum structure function through the high -energy electromagnetic probe and the deep non -bullet scattering of the nuclear, and the second is to produce strong through high -energy collisions to produce strong collisions Sub -state and excitement, study strong sublates.
my country's strong sub -structure research began in the theoretical research of layer model in the 1960s. Thanks to the great promotion of the Study Spectrum Experiment of the Starting Electronics Campaign in the 1990s, it is currently at the forefront of the world. Essence Among the important highlights of the year selected by Physics magazine edited by the American Institute of Physics in the past ten years, the two brightest results of strong interaction are: (1) The BESIII experiment group mainly based on Chinese scientists in 2013 discovered ZC (3900) Four Quicks; (2) The LHCB experiment team that Chinese scientists made outstanding contributions in 2015 discovered two PC five quarks. my country's theoretical physicists have made outstanding contributions in the predictions and interpretations of these Darks. They are invited to write relevant reviews for the top -level review journal of physics [39, 40]. The finding and theoretical research of these strange strong sub -state is the cutting -edge hotspot of strong sub -structural research, which further reveals the important role of non -quenching effects in the strong sub -structure and Quark's closed mechanism. We know from the history of material micro -structured research that the research of atomic spectrum has brought us the quantum theory of atoms and promoted the establishment of quantum mechanics; What new breakthrough discovery will the study of Qiangzi spectrum bring us? let us wait and see.
4.2 Nuclear structure under extreme conditions
Since the gate of Grefle has sorted out the element cycle table, people have been gradually discovering and creating new elements and nucleo (ie atomic nucleus). The nucleo that has been discovered at present includes more than 300 nuclein existing in nature and more than 3,000 individuals synthesized. The theory expects a total of about 9,000 nucleo. Therefore, there are still a large number of new nuclein waiting for people to explore, especially many theoretical model predictions that may exist at about 120 ultra -weight kernel stable islands. The classic nuclear shell structure model and collective motion model successfully explained the structure of the stabilized atomic nucleus observed in nature, but high -speed rotation, super deformed, abnormal neutron proton ratio, overweight, doping superzon and other extreme conditions There are many new phenomena and new structures in the nucleus. How to quantify the internal structure of all atomic nucleus under a unified theoretical framework is a new challenge facing the current nuclear structure physicist. The research on these unstable nuclear is also of great significance for understanding the process and abundance of nuclear celestial body physical fitness in the universe.
In addition to the nucleus composed of nucleus, people also tried to inject the superzi containing s quark in the atomic nucleus (
4.3 High -temperature and high -secret nuclear substance
The transformation of the state of material state dominated by strong interaction is essential to understand the evolution of the early universe and the structure and nature of the dense stars such as neutron stars. The material phase of the daily life is essentially dominated by electromagnetic interactions. We can get the corresponding phase diagram from experiments and theories. Similarly, the phase change of the substance with strong interaction is also described by its phase diagram (Figure 7). The substances of strong interaction include the following states:
(1) Nuclear Matter, a uniform system consisting of a large amount of mass and neutrons based on a certain density. At this time, the temperature is close to 0, and the corresponding heavy child chemical potential corresponding to the saturation density is about 900 MEV. The position of the nuclear center and the internal dense stars can be similar to the nuclear substance. In addition, nuclear substances may also be formed in heavy ion collision experiments.
(2) Hadron Gas. When heavy child chemistry is not large, and at the same time, it has a non -zero temperature, the strong son exists in a state similar to gas.
(3) Color SuperConductor. Increase the chemical momentum of heavy sub -chemistry, maintain low temperature, and strong interaction substances enter a color superconductor state, similar to electric superconductors at low temperature.
(4) Quark -gluonplasma (QGP). At high temperature and high dense, the quark that forms a strong son will mix the state of the plasma into a plasma, and be independent of each other.
Figure 7 QCD phase diagram. The horizontal axis is the chemical potential of heavy sub -(the number of heavy children or quarks), and the vertical axis is temperature
In the early stages of the universe, the positive and negative substances were the same, and the chemical potential of the heavy child was zero, but it had high energy. At that time, the strong interaction material was likely to be in the QGP state. At present, the temperature is gradually cooling, and the substance we see is in the state of nuclear matter. At present, the experimental research on QCD phase changes is mainly concentrated in two directions:
(1) Research on high temperature and low dense, ideal gas. The calculation of the QCD is found that when the chemical potential is small, as the system temperature rises, the system will transition from the strong child gas to the quark -gum plasma phase. The fluid dynamics simulation shows that QGP is closer to the ideal fluid. Experimental explorations in this direction are mainly carried out by the relatively strong ion collision (RHIC/BNL) of the Brook Haiwen National Laboratory and a large -scale strong child collision (LHC/CERN) at the European nuclear centers.
(2) Research on low temperature and high dense, color superconducting. This direction is mainly conducted by the German heavy ions research center (FAIR/GSI) and the heavy ion cooling storage ring (CSR) of the Institute of Modern Physics of the Lanzhou Chinese Academy of Sciences.
In addition, after the completion of the completion of the Institute of Modern Physics of the Chinese Academy of Sciences (HIAF) Development, nuclear security and nuclear technology application provides theoretical, methods, technology and talent support. 05
Summary and outlook
Strong interaction determines the structure of the micro -basic levels of Qiangzi and atomic nucleus, and it is also an important part of basic particles and cosmic celestial evolutionary physics. Although after about a hundred years of exploration, people have a comprehensive understanding of Qiang interaction, we still face many mysteries to be solved. The development of large scientific devices in China, including Beijing's positive and negative electronics collision (BEPCII), Hiaf, etc. The cooperation of theoretical and experiments has made the research of strong interaction physics in our country at the forefront of the world.
Note:
1) In 1925, the student of Luguford Blackt proved that the real process should be
2) https://www.nobelprize.org/prizes/physics/1927/wilson/biography ‐cal/
3) Zweig named it ACE.
4) As early as 1964, Bjorken and GLASHOW had a new quark corresponding to S Koko -Quark symmetrical guessing. At that time, people did not recognize its importance.
5) Now its name is j/ψ, it is because of it
references
[1] Kragh H. 2012, ARXIV: 1202.0954
[2] Rutherford E. Philosophical Magazine, 1899, 47: 109
[3] Rutherford E, Thomas R. Philosophical Magazine, 1909, 17: 281
[4] Rutherford E,Soddy F. The London,Edinburgh,and Dublin Philosophical Magazine and Journal of Science,1903,5(29):576
[5] Andrade e n
[6] Rutherford e. Phil. Mag. Ser. 6, 1911, 21: 669
[7] Rutherford E. The London, Edinburgh, and Dublin Philosophicalmagazine and Journal of Science, 1919, 37 (222): 581
[8] Rutherform E. Proceedings of the Royal Society A: Mathematical, Physical and Engineering Sciences, 1920, 97 (686): 374
[9] soddy f. Nature, 1913, 92: 399
[10] Aston F W. The London, Edinburgh, and Dublin Philosophonemagazine and Journal of Science, 1919, 38 (228): 707
[11] Bohr n. Nature, 1921, 107: 104
[12] Chadwick j. Nature, 1932, 129: 312
[13] hahn o. naturwissenschaften, 1939, 27: 11
[14] Meitner L, Frisch O. Nature, 1930, 143: 239
[15] Frisch o. Nature, 1930, 143: 276
[16] Blackett P. Journal of the Chemical Society Transactions, Seriesa, 1925, 107 (742): 349: 349
[17] IWANENKO D. Nature, 1934, 133: 981
[18] yukawa h. Proc. Phys. Math. Soc. Jap., 1935, 17: 48
[19] Choudhuri B, Bose D. Nature, 1941, 148: 259
[20] choudhuri b, bose d. Nature, 1942, 149: 302
[21] Lattes C, Muirhead H, Occhialini get al. Nature, 1947, 159: 694 [22] Mayer m g. Phys. Rev., 1948, 74: 235
[23] Mayer M g. PHYS. Rev., 1949, 75: 1969
[24] haxel o, jensen h, suess h e. Phys. Rev., 1949, 75 (11): 1766
[25] Rainwater J. Phys. Rev., 1950, 79: 432
[26] BOHR A. Phys. Rev., 1951, 81: 134
[27] Bohr a, mottelson b r. Phys. Rev., 1953, 89: 316
[28] BOHR A, Mottelson b r. Phys. Rev., 1953, 90: 717
[29] Bethe H A. PHYS. Rev., 1939, 55: 434
[30] Burbidge M E, Burbidge G, Fowler W Aet Al. Rev. Mod.phys., 1957, 29: 547
[31] HOFSTADTER R. Rev. Mod. PHYS., 1956, 28: 214
[32] ROOS M. Rev. Mod. Phys., 1963, 35: 314
[33] Aubert Jet Al. Phys. Rev. Lett., 1974, 33: 1404
[34] Augustin J Eet Al. Phys. Rev. Lett., 1974, 33: 1406
[35] Bloom E Det Al. Phys. Rev. Lett., 1969, 23: 930
[36] Breidenbach Met Al. Phys. Rev. Lett., 1969, 23: 935
[37] POLITZER H D. PHYS. Rev. Lett., 1973, 30: 1346
[38] gross d j, wilczek f. Phys. Rev. Lett., 1973, 30: 1343
[39] GUO F K, Hanhart C, Meißner Uet Al. Rev. Mod. PHYS., 2018, 90 (1): 015004
[40] Chen h x, chenw, liu xet al. Phys. REPT., 2016,639: 1
Reprinted content only represents the author's point of view
Does not represent the position of the Institute of Physics of the Chinese Academy of Sciences
If you need to reprint, please contact the original public account
Original title: Strong Interaction Nobel Prize Monastery
Source: China Physical Society Journal Network
Edit: Old man
- END -
The first China Western Western Science and Technology Innovation Contest was launched in Chengdu in 2022
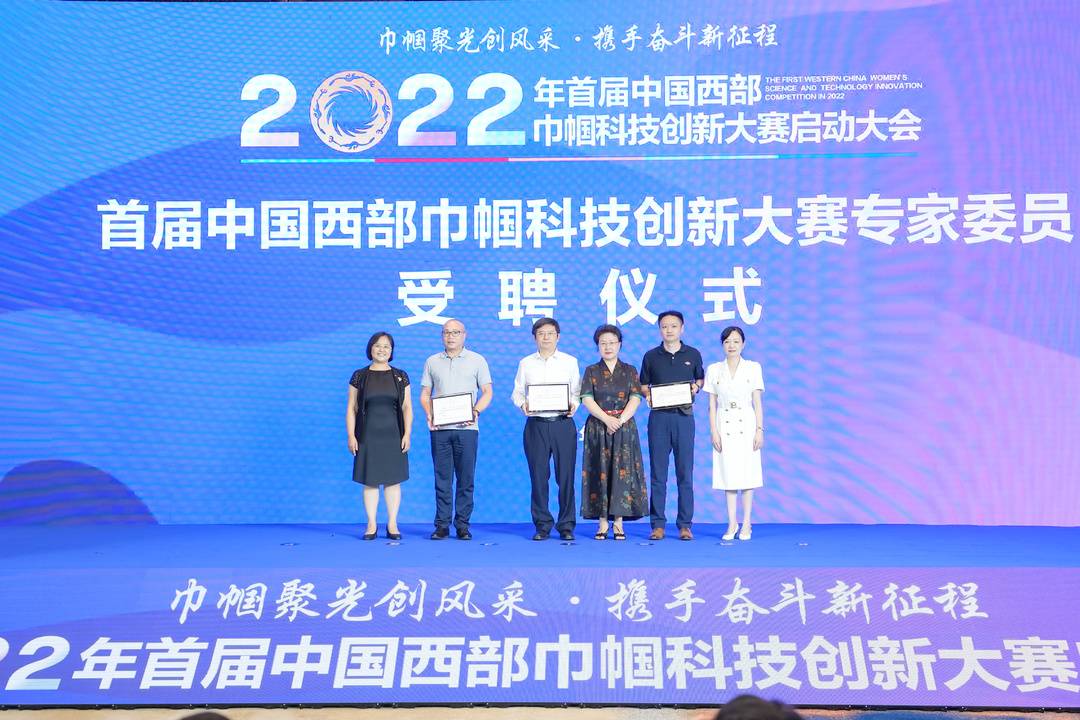
Cover news reporter Yang Ruiwen Photography Reporting part of the picture was prov...
Guests of the 5th China -Africa Media Cooperation Forum inspect Beijing Enterprise
On the morning of August 26, 2022, guests at the Fifth China -Africa Media Cooperation Forum inspected the Sida Times Group and Jingdong Group. More than 20 African African ambassadors, including the